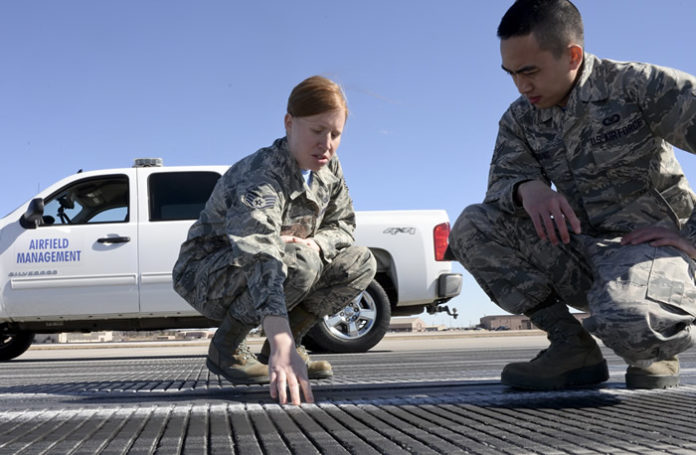
This article is a version of a paper included in the peer-reviewed proceedings from the GAP 2019 transportation engineering conference, which was managed by Geosynthetica’s publisher Minerva TRI. Here, W. Jeremy Robinson, P.E. of the US Army Engineer Research and Development Center (ERDC) writes on how ERDC has reviewed airfield pavement condition data. The tests described and the resulting data presented herein provide insight into PCC and asphalt pavement installations, weathering, cracking trends, and much more. Most of the research cited was sponsored by the Federal Aviation Administration and performed by ERDC. Permission was granted to the author by the Director, Geotechnical and Structures Laboratory, to publish this information.
ABOUT THE FEATURE IMAGE: Staff Sgt. Crystal Bennoch, 28th Operations Support Squadron NCO in-charge of airfield management operations, trains Airman 1st Class Justin Chung, 28th OSS airfield management coordinator, on properly inspecting the runway centerline markings at Ellsworth Air Force Base, S.D. This is a U.S. Air Force photo by Senior Airman Anania Tekurio/Released.
INTRODUCTION
Both airfield and highway pavements are a significant financial investment that, without periodic maintenance, will not achieve anticipated service life. The American Society of Civil Engineers (ASCE) publishes a national infrastructure report card every four years that uses a letter grade format to evaluate the overall condition of the nation’s infrastructure. In 2017, ASCE reported that the nation’s aviation system (i.e., airport facilities in general) was assigned an overall grade of D and that $157 billion was needed to address funding needs (ASCE, 2017).
The ability to forecast required maintenance and delay or mitigate pavement deterioration through improved material selection techniques increases the effective use of limited budgets.
ALSO FROM GAP: Geosynthetics in Diverse Railroad Applications
In order to achieve this goal, a standardized, repeatable pavement evaluation approach should be employed; and historical data should be reviewed to determine prevalent distress types and mechanisms. Further, these data should be used to direct research initiatives geared at addressing global issues that, if successful, motivate innovative design and maintenance techniques. This article presents the results of an effort to compile historical pavement condition data spanning up to 50 years from selected Army airfields for the primary purpose of assessing global pavement deterioration trends and identifying common pavement distress types. The data provided a means to recommend potential preventative maintenance solutions and fundamental material changes that should be investigated.
PAVEMENT CONDITION DATA BACKGROUND
A review of available historical reports conducted for this paper indicates that pavement condition surveys of Army airfields and structural evaluations were initiated in the late 1940s and early 1950s. Condition evaluations were performed by local field personnel identifying pavement distresses and providing an overall descriptive classification of pavement condition. In early reports, general descriptions such as “good, fair, poor, failed” were used to describe pavement condition; and few detailed measurements were performed. Structural evaluations consisted of extensive field investigation generally completed through direct-sampling techniques such as test pits and subsequent laboratory characterization and evaluation. These general procedures were followed through the 1970s.
In May 1982, the Department of the Army initiated a program to determine and evaluate the physical properties, load-carrying capacity, and general condition of the pavements at major U.S. Army airfields. This procedure detailed specific pavement distresses, defined methods for physical measurement of each distress, and defined severity levels of each identified distress. The resulting findings are used to calculate an overall Pavement Condition Index (PCI). PCI is a numerical indicator of pavement condition assigned to the following ratings: Good (100-86), Satisfactory (85-71), Fair (70-56), Poor (55-41), Very Poor (40-26), Serious (25-11), and Failed (10-0). Additionally, structural evaluations of airfield pavements became less labor intensive through the use of nondestructive falling and heavy-weight deflectometer (FWD and HWD) devices, thus providing for more data to be collected in less time with greater pavement area coverage. The standard method for performing airfield condition surveys to identify and quantify pavement distress (type, severity, and magnitude) is outlined in ASTM D5340 (ASTM, 2018), and the Department of Defense guidance for conducting a structural evaluation is detailed in UFC 3-260-03 (Headquarters, 2001). To provide repeatability in subsequent pavement evaluations, the entire airfield pavement facility is subdivided into smaller sample sections that are grouped by unique properties such as pavement surface type, pavement thickness, anticipated traffic, and/or repair and construction history. Delineation of unique pavement areas allows for repeated testing and inspection of the same portion of pavement, thus creating a history of pavement condition and deterioration.
Initiation of a specific and standardized evaluation method allowed for generally repeatable and reliable assessment of airfield pavements. The development of the PAVER software package in the late 1970s provided an electronic means of storing and summarizing all the field-collected data.
PAVEMENT STUDY OBJECTIVE AND APPROACH
The main objective of the study was to compile historical condition data (e.g., PCI) and evaluate the general relationship between airfield pavement condition and pavement age. The data were used to observe prevalent trends and identify predominant distress mechanisms to make recommendations for maintenance techniques or potential material changes to extend pavement performance.
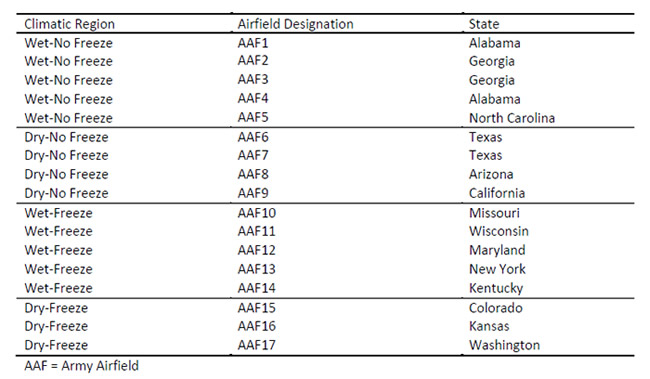
Seventeen Army airfields were selected for initial review (Table 1). These were located within the continental United States and represented a cross section of airfield pavements throughout four climatic regions: (1) wet-no freeze, (2) wet-freeze, (3) dry-no freeze, and (4) dry-freeze. Data were selected from multiple climatic regions to limit potential bias introduced by selecting a majority of airfield pavements from a single region. It should be noted that it was not the intent of this study to delineate deterioration based on climatic region but rather to observe global deterioration trends and failure mechanisms.
From each airfield, multiple sections were selected, representing both asphalt and portland cement concrete pavements. PCI and construction history data were collected for each section. Pavement age was based on available construction history and plotted with a PCI number to observe general trends.
PAVEMENT CONDITION DATA RESULTS
Overall Trends
Figure 1 presents all data points collected for AC pavements. The data shown in this plot represent 91 distinct AC pavement sections. While there is significant scatter in the data, it is observed that most AC pavements experience a generally steep decline in condition from initial construction to age 20, which is the current design life with most AC pavements at or older than 30 years having PCI values that are considered poor to serious (PCI ranging from 55 to 10).
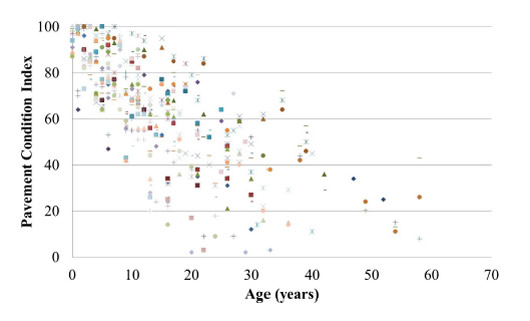
Data values collected for 59 distinct PCC pavement sections are shown in Figure 2. In general, the trend between PCI and pavement age is not as clear as that for asphalt pavements; however, a more gradual decrease in PCI over time can be observed. Reported PCI values become much more variable as the pavement age increases, a trend which could be a function of maintenance (or the lack thereof) or drastic changes in airfield operations. Condition values of the older pavements ranged from good to failed, and it is noted that some PCC pavements have been in-service for well over 40 years with satisfactory pavement condition ratings.
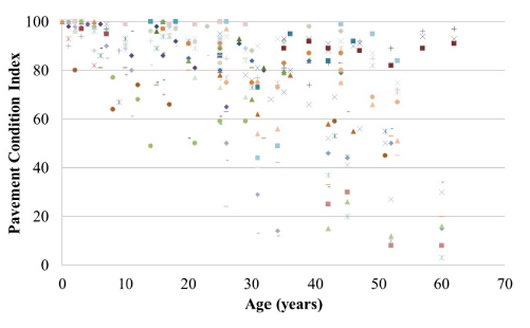
Review of the plotted PCI for each pavement type generally follows expected trends. Asphalt pavements were found to experience fairly rapid deterioration in condition, and PCC pavements experienced a more gradual decrease in condition. Although the compiled data include maintenance activities, which can increase PCI, these figures provide an indication of the overall deterioration trends.
After review of the overall data collection, thirteen pavement sections, summarized in Table 2, were selected for further data collection and analysis. Seven PCC pavement sections were selected with ages ranging from 15 to 56 years, and six AC pavement sections were selected with ages ranging from 4 to 45 years. Note that some sections are repeated in the table of selected asphalt pavements with varying ages. This is due to some major rehabilitation (mill/overlay) and/or reconstruction activity, which was considered to reset the age (in terms of surface condition) of the pavement.
Distress mechanisms were identified by type (climate/durability, load, and other) to evaluate trends over time. Most distress types have three levels of severity: low, medium, and high. Severity level is based on the size of the distress and/or its potential to produce Foreign Object Debris (FOD). A detailed explanation of distress classification and severity level can be found in ASTM D5340 (ASTM, 2018).
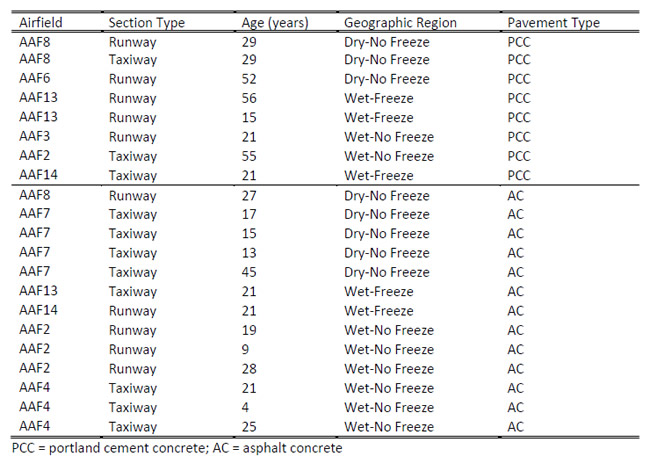
PCC Pavement Condition Index
Each PCC distress type, as defined in ASTM D5340 (ASTM, 2018), is organized in Table 3 by distress mechanism. Figure 3 displays the contribution of each distress mechanism associated with various ages for the PCC pavements. The data presented represent a compilation of all selected PCC pavements and the associated distress contribution observed for each evaluation. The data indicate that the initial types of distress occurring in PCC pavements are identified as climate/durability and other. These distresses generally occur early in the pavement life (from 0 to approximately 15 years). Load-related distresses generally start to occur around 16 years and trend upward to peak in the 40-year range. Note that one pavement section showed load-related distress one year after construction, an apparent exception to the general trend. After 40 years of pavement age, the load-related distress mechanism experiences a downward trend, which is likely due to maintenance operations that typically consist of total slab replacement if distresses are considered medium to high severity.
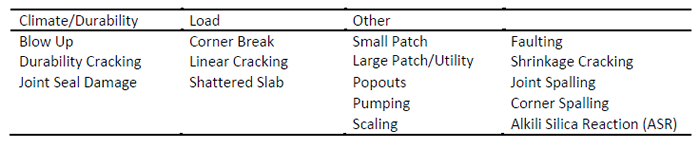
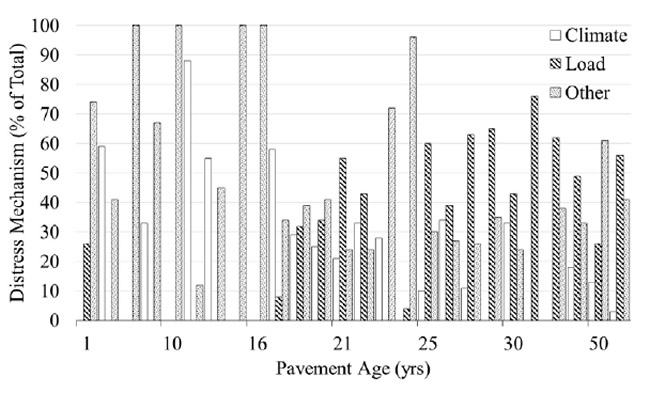
Table 4 shows an example PCI deterioration graph with pavement age and typical distress mechanisms for a PCC pavement section (AAF8 Runway is shown). Other PCC pavements displayed similar trends and distress types. It was found that joint and corner spalling were recurring distress types and were first documented from 2 to 6 years after initial construction. In general, joint and corner spall severity was found to progress to a higher severity level (i.e., low to medium or medium to high) over a period of approximately 4 years. Joint seal damage was also identified as a common distress across all PCC pavements and was first observed 2 to 4 years after construction. Distress severity level was found to increase after a time span of approximately 9 to 10 years.
For PCC pavements, note that repair activities (i.e., spall repair or crack sealing) are measured as pavement defects. This results in the PCC pavements’ never returning to a PCI of 100. However, review of the data indicates that the rate of deterioration of most PCC pavements was gradual in nature.
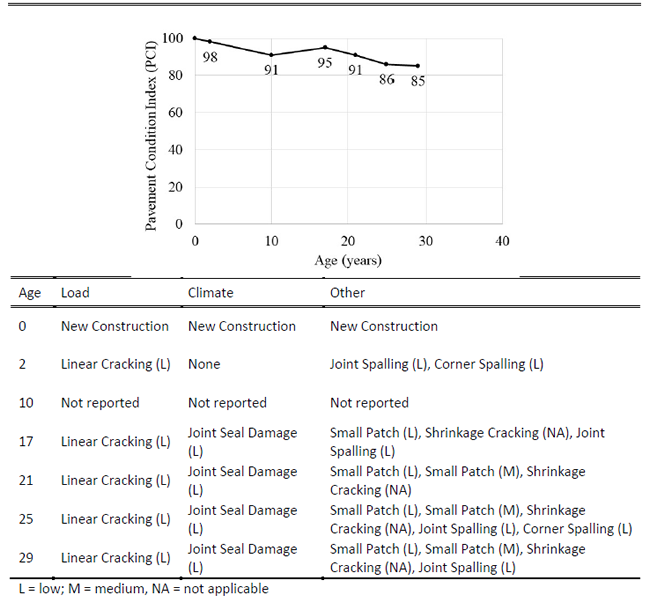
Asphalt Pavement Condition Index
Each asphalt distress type, as defined in ASTM D5340 (ASTM, 2018), is organized in Table 5 by distress mechanism. Distress mechanism with pavement age is presented graphically in Figure 4.The data presented represent a compilation of all selected AC pavements and the associated distress contribution observed for each evaluation. Review of the selected data indicates that climate-related distresses are the primary contributor to PCI reduction for the first 10 to 12 years of service life and remain a major contributor for most of the identified pavement age. Load-induced distress mechanisms were found to generally begin in the 14- to 15-year age range and peak at the 20- to 21-year age range. A decrease in load-related distress was noted after approximately 20 years, likely due to some form of major rehabilitation.
A review of historical data indicates relationships between asphalt pavement age and surface deterioration (PCI) are more difficult to define. It was found that repair and/or maintenance activities tend to occur frequently, in turn causing multiple increases in condition index values. In general, the data indicate asphalt-surfaced pavements do not approach a 20-year life with regards to PCI without major maintenance.
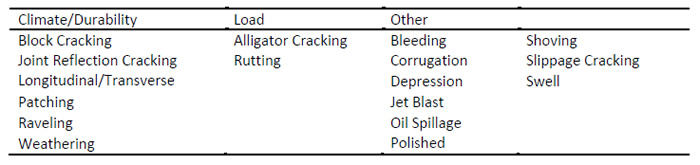
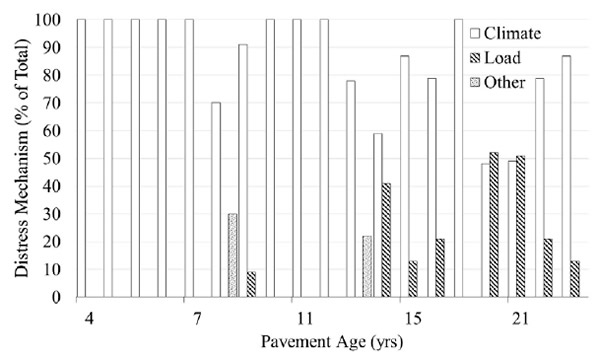
Table 6 shows an example PCI deterioration graph with pavement age and typical distress mechanisms for an asphalt pavement section (AAF7 Taxiway is shown).
Asphalt overlays will return the PCI to a value of near 100, essentially resetting the deterioration curve, as can be seen at year 17 and 32 in Table 6. However, underlying distresses (e.g., fatigue cracking, rutting, or loss of subgrade support due to rapid changes in subgrade moisture content from poor drainage or utility leaks) may increase the rate of distress seen after maintenance activities.
Longitudinal and transverse cracking were found to be the most common and recurring AC pavement distresses, typically first observed in the 2- to 4-year time frame after construction or rehabilitation. An increase in severity level was commonly observed over an 8- to 10-year time period. Weathering was also a common distress type, generally identified 9 to 10 years after construction.
OBSERVATIONS FROM PCI TRENDS
It was observed from both PCC and AC datasets that non-load related distresses are the primary contributor to pavement deterioration, particularly early in pavement life. Current maintenance practices are generally reactive in nature; however, the results suggest that preventative techniques or material changes should be pursued to mitigate or reduce these distresses on airfield pavements.
One example is the application of a pavement sealer to reduce or mitigate the potential for weathering on asphalt pavements. Research (Rushing et al, 2015) has shown that pavement preservation techniques, such as surface treatments, can be used in low-speed airfield applications such as aprons, taxiways, or helipads. Further, Rushing et al. (Rushing et al, 2015) concluded that some surface treatments are effective in retarding longitudinal/transverse cracking.
Another option to mitigate climate-related distress on asphalt pavements, particular cracking, takes a more fundamental approach. Early-age cracking can indicate that current airfield asphalt mix design procedures do not supply enough asphalt binder to the mix to maintain flexibility and resist cracking.
The highway paving industry is investigating balanced mix design procedures, in which optimum asphalt binder content is selected based on a balance between rutting performance and cracking performance, although a definitive laboratory cracking test is still needed. Al-Qadi et al. (Al-Qadi et al, 2015) developed the semi-circular bend (SCB) Illinois Flexibility Index Test (I-FIT), which is a laboratory cracking test, to evaluate asphalt mixture brittleness. The research determined that mixtures with a flexibility index (FI) less than 2 were the worst performers, and mixtures with an FI value greater than 6 were the best performers. Bennert et al. (Bennert et al, 2017) applied similar methodology to perform a forensic investigation of pavements on the JFK and Newark International airport runways. Pavements with FI values greater than 6 were found to have little to no observed cracking, whereas poor cracking performance was observed in pavements with FI values less than 4. A similar approach could be used for military airfield asphalt pavements, since the data do not indicate that load-related distresses are a widespread problem.
A similar approach could be pursued for PCC pavements. Poole and Rollings (Poole and Rollings, 2009) suggested that in-place air content and air content variability could be a contributor to joint spalling and that research was needed to investigate differences between air content of fresh concrete and hardened concrete. It was suggested that higher air contents in fresh concrete may be needed to account for lower in-place air content values. Further, Harrington et al. (Harrington et al, 2018) suggested that the air void spacing factor (i.e., the distance water must travel to reach an air void) impacts durability. Additionally, Poole and Rollings (Poole and Rollings, 2009) recommended that a detailed investigation into the role of deicing salts on aggregate durability be conducted.
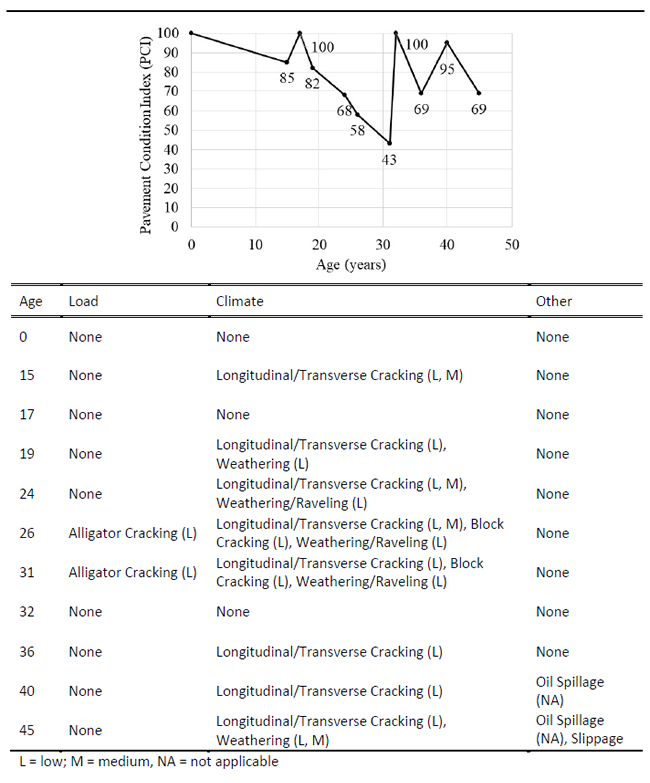
CONCLUSIONS
The initial pavement condition data collection effort consisted of collecting PCI data for 91 AC pavement sections and 59 PCC sections. Six AC pavement sections and seven PCC pavement sections were selected from the initial data collection effort for further analysis and evaluation. The following are the main findings from this study.
PCC Pavement Findings
- The dataset indicates that the selected PCC pavements generally should meet or exceed a 40- year PCI performance expectation.
- Non-load classified distresses were found to be the primary distress mechanism identified during the first 15 years. Load-related distresses were found to occur first around year 16 and trend upward to peak at year 40. A decrease in load-related distresses was observed after year 40, likely due to maintenance operations.
- Joint and corner spalling was found to be a recurring distress identified and e first documented from 2 to 6 years after initial construction. In general, the distress severity increased to a higher level over a period of approximately 4 years.
- Joint seal damage was found to be a common distress across the selected PCC pavements and was generally identified 2 to 4 years after construction. The distress severity level generally increased after 9 to 10 years.
Asphalt Pavement Findings
- Observed PCI trends indicate that the selected AC pavements do not approach a 20-year PCI age without some form of major rehabilitation (overlay or mill/overlay).
- Climate-related distresses were found to be the primary distress mechanism for the first 10 to 12 years and remained a major contributor for most of the pavement age.
- Load-related distresses in AC pavements were found to begin generally in the 14- to 15-year pavement age range and peak around 20 years. A decrease in load distresses was observed after 20 years, likely due to rehabilitation.
- Longitudinal/transverse cracking was found to be a recurring AC pavement distress, generally first observed in the 2- to 4-year time frame. An increase in severity level was observed over an 8- to 10-year time period.
- Weathering was also a common climate distress type, generally identified 9 to 10 years after construction.
ACKNOWLEDGEMENTS
The tests described and the resulting data presented herein, unless otherwise noted, were obtained from research sponsored by the Federal Aviation Administration and performed by the U.S. Army Engineer Research and Development Center. Permission was granted by the Director, Geotechnical and Structures Laboratory, to publish this information.
ABOUT THE AUTHOR
- Jeremy Robinson, P.E. works for the U.S. Army Engineer Research and Development Center (ERDC). Learn more about ERDC’s work at https://www.erdc.usace.army.mil/.
REFERENCES
Al-Qadi, I., H. Ozer, J. Lambros, A. El Khatib, P. Singhvi, T. Khan, J. Riveria-Periz, and B. Doll. (2015). Testing Protocols to Ensure Performance of High Asphalt Binder Replacement Mixes Using RAP and RAS. Research Report No. FHWA-ICT-15-017. Rantoul, IL: Illinois Center for Transportation.
American Society for Testing and Materials (ASTM). (2018). Standard Test Method for Airport Pavement Condition Index Surveys. Designation: D 5340-12, West Conshohocken, PA: ASTM International.
American Society of Civil Engineers (ASCE). (2017). ASCE’s 2017 Infrastructure Report Card. Washington DC. Accessed May 2018. Retrieved from https://www.infrastructurereportcard.org/.
Bennert, T., C. Ericson, R. Shamborovskyy, and C. Bognacki. (2017). “Moving towards Asphalt Binder and Mixture Protocols to Minimize Fatigue Cracking on Asphalt Airfields.” Transportation Research Record: Journal of the Transportation Research Board, 2633: 117-126.
Harrington, D., M. Ayers, T. Cackler, G. Fick, D. Schwartz, K. Smith, M.B. Snyder, and T.V. Dam. (2018). Guide for Concrete Pavement Distress Assessments and Solutions: Identification, Causes, Prevention and Repair. Ames, IA: National Concrete Pavement Technology Center, Iowa State University.
Headquarters, Departments of the Army, the Navy, and the Air Force. (2001). Airfield Pavement Evaluation. Unified Facilities Criteria 3-260-03. Washington, DC: Headquarters, Departments of the Army, the Navy, and the Air Force.
Poole, T. S., and R. S. Rollings. (2009). Investigation of Sliver-Spall Damage at Offutt Air Force Base, Nebraska. Technical Report ERDC/GSL TR-09-6. Vicksburg, MS: U.S. Army Engineer Research and Development Center.
Rushing, J., J. Falls, and B. Andrews. (2015). Asphalt Surface Treatments’ Impact on Pavement Condition at Army Airfields after Five Years. Technical Report ERDC/GSL TR-15-6. Vicksburg, MS: U.S. Army Engineer Research and Development Center.